Revision as of 18:37, 30 March 2017 edit88.105.39.101 (talk) Undid revision 773008629 by Quasar G. (talk) Please do not blindly remove someone edits, please start a discussion on the talk page. The content removed, cited some generic volume of regulations.← Previous edit | Revision as of 18:45, 30 March 2017 edit undoQuasar G. (talk | contribs)Extended confirmed users, Pending changes reviewers, Rollbackers6,685 edits Reverted to revision 773008629 by Quasar G. (talk): Reverting PURELY to fix the reference errors, will discuss on talk. (TW)Next edit → | ||
Line 1: | Line 1: | ||
⚫ | '''Minimum control speeds (V<sub>MC</sub>s)''' are so-called ] that are included in the limitations section of airplane flight manuals (AFM) of all ]. In general, a minimum control speed is the ] below which ] or ] control of an airplane (i.e. the desired heading or bank angle) on the ground (runway) or in the air can no longer be maintained by the pilot after failure of a wing-mounted engine, or while such an engine is inoperative, as long as the thrust of the opposite engine on the other wing is at the maximum (takeoff) setting. V<sub>MC</sub>s are also used by airplane design engineers for sizing the ] or stabilizer and the aerodynamic ]. | ||
{{Cleanup|reason=This article while containing much factual information, is also an opinion piece written or sourced from one author. It could do with some work|date=March 2017}} | |||
Many manuals for pilots and reports by accident investigators present and use V<sub>MC</sub>s as they are defined in ] that are for design and certification of multi-engine airplanes, such as FAR 23 or FAR 25 or equivalent<ref name=FAR23>{{cite web|last=Federal Aviation Administration, USA.|title=Federal Aviation Regulations (FAR)|url=http://www.ecfr.gov/cgi-bin/text-idx?gp=&SID=3a49096c5e53bff7eae8902299a5a6aa&mc=true&tpl=/ecfrbrowse/Title14/14CIsubchapC.tpl|work= Part 23 and Part 25, § 149|accessdate=May 15, 2016}}</ref><ref name=CS23>{{cite web|last=European Aviation Safety Agency|title=Certification Specifications (CS)|url=http://easa.europa.eu/agency-measures/certification-specifications.php|work=CS-23 and CS-25, § 149|accessdate=Oct 28, 2013}}</ref> and not as they apply to the operational use of the airplanes by pilots. Therefore, this article intends to bridge the knowledge gap between design engineers, flight-test crews, multi-engine rated (airline) pilots, and airplane accident investigators, by explaining the minimum control speeds V<sub>MC</sub> as taught by aeronautical universities and used in airplane design<ref name=Ros1>{{cite book|last=Roskam|first=Dr. Jan|title=Airplane Design Part VII. Lateral-Directional Stability and Control Characteristics for Steady Straight Line Flight, Chapter 5|year=1989|publisher=DARcorporation|page=286|url=https://shop.darcorp.com/index.php?route=product/category&path=60 | |||
}}</ref><ref name=Ros2>{{cite book|last=Roskam|first=Dr. Jan|title=Airplane Design Part II. Preliminary Configuration Design and Integration of the Propulsion System, Chapter 11|year=1997|publisher=DARcorporation|page=267|url=https://shop.darcorp.com/index.php?route=product/category&path=60 | |||
⚫ | '''Minimum control speeds (V<sub>MC</sub>s)''' are so-called ] that are |
||
}}</ref> and by (experimental) ]s, such as the ], the ] and the ].<ref name=USAFTPS>{{cite book|last=USAF Test Pilot School, Edwards Air Force Base, CA, USA|title=Engine-Out Theory, Chapter 11|year=1992|url=http://www.dtic.mil/dtic/tr/fulltext/u2/a319982.pdf | |||
|accessdate=May 15, 2016}}</ref><ref name=ETPS>{{cite book|last=Empire Test Pilots' School, Boscombe Down, UK.|title=Flight on Asymmetric Power}}</ref><ref name=USNTPS>{{cite book|last=USNaval Test Pilot School|title=Flight Test Manual USNTPS-FTM-No. 103, Fixed Wing Stability And Control, Theory and Flight Test Techniques, Chapter 6 – Asymmetric Power Flying Qualities|url=http://www.usntpsalumni.com/Resources/Documents/USNTPS_FTM_103.pdf | |||
They are typically established as part of an aircraft certification process, and can be expected to be listed in the aircraft flight manuals (AFM). | |||
|accessdate=May 15, 2016}}</ref> | |||
== Regulatory minimum control speeds V<sub>MC</sub> == | == Regulatory minimum control speeds V<sub>MC</sub> == | ||
]Aviation regulations (such as FAR and EASA CS parts 23 and 25 and equivalent)<ref> |
]Aviation regulations (such as FAR and EASA CS parts 23 and 25 and equivalent)<ref name=FAR23 /><ref name=CS23 /> define several different V<sub>MC</sub>’s and require design engineers to size the vertical tail and the aerodynamic ] of the airplane to comply with these regulations. The minimum control speed airborne (or in the air - V<sub>MCA</sub>) is the most important minimum control speed of a multi-engine airplane. In many aviation regulations and AFMs, V<sub>MCA</sub> is listed as V<sub>MC</sub> though,<ref name=FAR23 /><ref name=CS23 /> with the addition that V<sub>MC</sub> is the minimum control speed for the takeoff configuration. However, during other flight phases, such as continued takeoff after flap retraction, cruise flight and approach for landing when the flaps are still up, a V<sub>MCA</sub> applies as well. | ||
Therefore most test pilot schools teach to use V<sub>MCA</sub>, rather than V<sub>MC</sub>, and include the airplane configuration or flight phase with it. Other defined V<sub>MC</sub>s are minimum control speed on the ground (V<sub>MCG</sub>) and minimum control speed during approach and landing (V<sub>MCL</sub>). In addition, on four or more engine airplanes, V<sub>MC</sub>s exist for cases with either one or two engines inoperative (on the same wing). Figure 1 illustrates the V<sub>MC</sub>s that are defined in the applicable civil aviation regulations<ref name=FAR23 /><ref name=CS23 /> and in military specifications.<ref name=MILSPEC>{{cite book|last=Military Specification MIL-F-8785C, superseded by MIL-STD-1797|title=Flying Qualities of Piloted Airplanes}}</ref> | |||
The following table lists |
The following table lists all V<sub>MC</sub>s. To avoid misunderstanding, this article uses V<sub>MC(A)</sub> when either V<sub>MCA</sub> or V<sub>MC</sub> for a flight phase is meant. | ||
{| class="wikitable" | {| class="wikitable" | ||
|- | |- | ||
! Type V<sub>MC</sub> !! Description | ! Type V<sub>MC</sub> !! Description (for pilots) | ||
|- valign="top" | |- valign="top" | ||
| V<sub>MC</sub>|| minimum control speed (representing all V<sub>MC</sub>‘s). In aviation regulations<ref name=FAR23 /><ref name=CS23 /> and many manuals used as V<sub>MCA</sub> for the takeoff configuration (takeoff flaps selected). In this article V<sub>MC(A)</sub> is used to denote V<sub>MC</sub> in the air. | |||
| V<sub>MC</sub>|| Minimum control speed. | |||
|- valign="top" | |- valign="top" | ||
| V<sub>MCG</sub>|| Minimum control speed on the ground (takeoff run). | | V<sub>MCG</sub>|| Minimum control speed on the ground (takeoff run). | ||
Line 106: | Line 107: | ||
The published V<sub>MC(A)</sub> is a standardized V<sub>MC(A)</sub>, refer to a few of the test conditions mentioned above and in applicable papers.<ref name=Horl2>{{cite web|last=Horlings|first=Harry|title=Control and Performance during Asymmetrical Powered Flight|url=http://www.avioconsult.com/downloads/Control%20and%20Performance%20During%20Asymmetrical%20Powered%20Flight.pdf|publisher=AvioConsult|accessdate=Oct 28, 2013}}</ref> Measuring all V<sub>MC</sub>s of an airplane for all configurations would leave pilots with huge data manuals. Publishing a worst case, standardized V<sub>MC(A)</sub> is always safe, provided the pilot is aware of the applicable limitations for the standardized V<sub>MC(A)</sub> to be valid. | The published V<sub>MC(A)</sub> is a standardized V<sub>MC(A)</sub>, refer to a few of the test conditions mentioned above and in applicable papers.<ref name=Horl2>{{cite web|last=Horlings|first=Harry|title=Control and Performance during Asymmetrical Powered Flight|url=http://www.avioconsult.com/downloads/Control%20and%20Performance%20During%20Asymmetrical%20Powered%20Flight.pdf|publisher=AvioConsult|accessdate=Oct 28, 2013}}</ref> Measuring all V<sub>MC</sub>s of an airplane for all configurations would leave pilots with huge data manuals. Publishing a worst case, standardized V<sub>MC(A)</sub> is always safe, provided the pilot is aware of the applicable limitations for the standardized V<sub>MC(A)</sub> to be valid. | ||
=== Definition of V<sub>MC(A)</sub> === | |||
{| class="wikitable" | |||
|- | |||
! '''For airplane design engineers:''' !! '''For pilots:''' | |||
|- valign="top" | |||
| FAR and EASA/CS 23 and 25 § 149<ref name=FAR23 /><ref name=CS23 /> and equivalent present the definition of V<sub>MC</sub> (is V<sub>MCA</sub>) for the design and certification of multi-engine airplanes: | |||
''Minimum control speed is the minimum flight speed at which the airplane is controllable with a bank angle of not more than 5° when the critical engine suddenly becomes inoperative and the remaining engine is operating at takeoff power.'' | |||
This definition is correct for tail design engineers and for certification but is often inappropriately copied into pilot manuals, because it contains elements that are neither appropriate, nor applicable to operational pilots. | |||
| Once the airplane is designed and built, the selected tail size imposes control limitations on, i.e. conditions to, pilots. The V<sub>MC(A)</sub> definition for use by pilots is therefore different from the V<sub>MC(A)</sub> definition for tail design engineers and for certification, and could be: | |||
{{color|blue|''V<sub>MC(A)</sub> is the minimum speed for maintaining control after a sudden engine failure and for maintaining steady straight flight while an engine is inoperative when the corresponding opposite engine is providing maximum thrust, provided a bank angle is being maintained of (3° – ) 5° away from the inoperative engine and the rudder is used up to maximum to maintain steady straight flight.''}} | |||
The exact required bank angle (also used to size the vertical tail) is to be provided by the airplane manufacturer with the V<sub>MC</sub> data. | |||
In addition, the manufacturer should specify the configuration (flaps, etc.) for which this, and other published V<sub>MC</sub>s is valid. | |||
V<sub>MC(A)</sub> is defined for steady straight flight only, while banking a few degrees away from the inoperative engine and not for turns. | |||
|} | |||
=== Display of V<sub>MC(A)</sub> === | === Display of V<sub>MC(A)</sub> === |
Revision as of 18:45, 30 March 2017
Minimum control speeds (VMCs) are so-called V-speeds that are included in the limitations section of airplane flight manuals (AFM) of all multi-engine airplanes. In general, a minimum control speed is the calibrated airspeed below which directional or lateral control of an airplane (i.e. the desired heading or bank angle) on the ground (runway) or in the air can no longer be maintained by the pilot after failure of a wing-mounted engine, or while such an engine is inoperative, as long as the thrust of the opposite engine on the other wing is at the maximum (takeoff) setting. VMCs are also used by airplane design engineers for sizing the vertical tail or stabilizer and the aerodynamic flight control surfaces.
Many manuals for pilots and reports by accident investigators present and use VMCs as they are defined in aviation regulations that are for design and certification of multi-engine airplanes, such as FAR 23 or FAR 25 or equivalent and not as they apply to the operational use of the airplanes by pilots. Therefore, this article intends to bridge the knowledge gap between design engineers, flight-test crews, multi-engine rated (airline) pilots, and airplane accident investigators, by explaining the minimum control speeds VMC as taught by aeronautical universities and used in airplane design and by (experimental) test pilot schools, such as the USAF Test Pilot School, the Empire Test Pilot's School and the US Naval Test Pilot School.
Regulatory minimum control speeds VMC

Aviation regulations (such as FAR and EASA CS parts 23 and 25 and equivalent) define several different VMC’s and require design engineers to size the vertical tail and the aerodynamic flight controls of the airplane to comply with these regulations. The minimum control speed airborne (or in the air - VMCA) is the most important minimum control speed of a multi-engine airplane. In many aviation regulations and AFMs, VMCA is listed as VMC though, with the addition that VMC is the minimum control speed for the takeoff configuration. However, during other flight phases, such as continued takeoff after flap retraction, cruise flight and approach for landing when the flaps are still up, a VMCA applies as well.
Therefore most test pilot schools teach to use VMCA, rather than VMC, and include the airplane configuration or flight phase with it. Other defined VMCs are minimum control speed on the ground (VMCG) and minimum control speed during approach and landing (VMCL). In addition, on four or more engine airplanes, VMCs exist for cases with either one or two engines inoperative (on the same wing). Figure 1 illustrates the VMCs that are defined in the applicable civil aviation regulations and in military specifications. The following table lists all VMCs. To avoid misunderstanding, this article uses VMC(A) when either VMCA or VMC for a flight phase is meant.
Type VMC | Description (for pilots) |
---|---|
VMC | minimum control speed (representing all VMC‘s). In aviation regulations and many manuals used as VMCA for the takeoff configuration (takeoff flaps selected). In this article VMC(A) is used to denote VMC in the air. |
VMCG | Minimum control speed on the ground (takeoff run). |
VMCA | Minimum control speed airborne (or in the air), while one engine inoperative (n-1). In this article also presented as VMC(A). |
VMCL | Minimum control speed during approach and landing, while one engine inoperative (n-1). |
VMCA1 | Minimum control speed - airborne, while one engine inoperative (n-1, same as VMCA, used for four or more engine airplanes). |
VMCA2 | Minimum control speed - airborne while two engines inoperative (on the same wing, n-2, used for four or more engine airplanes). |
VMCL1 | Minimum control speed during approach and landing while one engine inoperative (n-1, same as VMCL, used for four- or more engine airplanes). |
VMCL2 | Minimum control speed during approach and landing while two engines inoperative (on the same wing, n-2, used for four- or more engine airplanes). |
Propulsion system malfunction - PSM
When an engine or, more appropriate, a propulsion system fails and the corresponding opposite engine is generating maximum thrust, the thrust distribution on the airplane has become asymmetrical, resulting in a large thrust yawing moment and a yaw rate in the direction of the failed engine. Instantaneously, a sideslip develops causing the total drag of the airplane to increase considerably and hence, the rate of climb or even the altitude to decrease. The rudder is the only aerodynamic control available to the pilot to counteract the asymmetrical thrust yawing moment, whether on the ground or in the air. In the air, banking (including roll side effect due to yawing) is controlled using the ailerons.

Following recovery to steady straight flight, a rudder side force remains required to balance the asymmetrical thrust. The rudder side force however, also causes the airplane to displace in the direction of the force, resulting in a sideslip to the left (into the dead engine, Figure 2). This sideslip, in turn, generates a side force due to sideslip, opposite of the rudder side force. The sideslip continues to increase until the increasing side force due to sideslip is equal to the rudder side force (that continues to be required to counteract the thrust yawing moment). Then the sum of the side forces is zero; a balance of side forces is established and the sideslip does not increase anymore. Hence, when keeping the wings level, a sideslip cannot be avoided. This sideslip results in a higher than minimum drag and a lower rate of climb. Below is explained how sideslip is reduced and the one engine inoperative climb performance is increased.
As stated in the introduction, when the thrust is asymmetrical there is an airspeed below which the generated rudder side force or the ailerons-generated lateral forces for the given fixed (designed) sizes of these control surfaces are not large enough to counteract the asymmetrical thrust yawing or rolling moments: the heading and the bank angle cannot be maintained below this speed, resulting in the loss of control; the airplane does not respond to the control inputs by the pilot anymore. This speed is the minimum control speed - airborne (VMC(A)). Loss of control is also addressed in the Aircraft upset article.
Minimum control speed airborne – VMC(A)
The vertical tail or vertical stabilizer of a multi-engine airplane plays a crucial role in maintaining directional control while an engine fails or is inoperative. Tail design engineers of multi-engine airplanes face the challenge of designing a tail as small and light-weight as possible (to save airplane weight and reduce production cost) while the tail should be capable of providing the required yawing moment to counteract the asymmetrical thrust yawing moment. A small vertical tail however, requires a higher minimum speed for maintaining control when the thrust is maximum asymmetrical, because the counteracting forces generated by rudder and ailerons are proportional to the square of the airspeed (V) and to the area of the aerodynamic control surfaces (S) – refer to the lift equation (L ≡ ½ρVSCLα). Hence, when the tail is small, VMC’s are high. VMC(A) is also used to calculate the takeoff speeds rotation speed VR and takeoff safety speed V2; refer to FAR or CS § 25.107. A high VMC(A) therefore results in higher takeoff speeds and hence, longer required runways (which operators do not like); the payload might have to be reduced for shorter runways. Aviation regulations (§ 149) limit the maximum approved VMC(A) to 1.2 times the stall speed VS, which results in the smallest allowable size of the vertical tail with rudder.
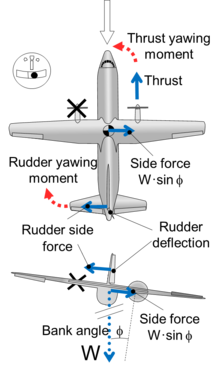
A large tail allows for a lower speed to still counteract the asymmetrical thrust, resulting in a lower VMC(A) but in increased airplane weight and production cost. A large tail, besides an increased weight, also causes adverse effects on controllability, such as increased slipstream effects. Hence, the design engineers try to keep the size of the rudder as small as possible.
There is a favorable influence on the balance of side forces that the tail design engineer is allowed to use for limiting the size of the vertical tail, which is a bank angle. Banking an airplane causes a component of the airplane weight (W - figure 3) to act as a side force in the center of gravity (c.g.) in the body-fixed y axis. This side force W•sin ø (airplane gross weight times the sine of bank angle phi) affects the balance of side forces that act on the airplane while an engine is inoperative and the wings are not level/horizontal. Aviation regulations (§ 149) allow the design engineer to use a bank angle of maximum 5° for sizing the vertical tail. This small bank angle, provided it is away from the inoperative engine, generates a side force that, like the side force due to sideslip as shown in figure 2, also counteracts the rudder side force that continues to be required to counteract the thrust yawing moment. Side force W•sin ø can reduce the sideslip angle to zero, therewith reducing the drag to a minimum, hence maximizing the remaining climb performance. This force acts in the c.g., hence its moment arm is zero; the small bank angle causes no adverse moments.
Therefore, the design engineer calculates the required size of the vertical tail using a regulations-approved bank angle of maximum 5° (away from the inoperative engine) which results in the smallest possible tail, and in the lowest airplane gross weight and production cost. Besides reducing the sideslip and drag, a small bank angle also reduces VMC(A), as is shown in the next and in the VMC(A) Testing paragraphs. The iterative tail design process, including the integration of the propulsion system, is presented by Dr. Jan Roskam, University of Kansas in his Airplane Design series of books.

The graph in figure 4 shows VMC(A) and the sideslip angle for different bank angles of a sample airplane after failure of the left engine (engine No. 1). The asymmetrical thrust setting and the rudder input are maximal, the other factors that have influence on VMC(A) are at their worst-case values (described in the next section). The graph is calculated using the data presented in the paper The Effect of Bank Angle and Weight on the Minimum Control Speed VMCA of an Engine-out Airplane
The airspeed that results from the bank angle for which the sideslip is zero is the VMCA (also incompletely called VMC) that is published in the AFM (in this example 87 knots). At bank angles larger than 6° away from the failed engine, for this sample airplane, the sideslip angle increases to 14°, which is the limit in the calculations to avoid fin stall due to increased sideslip. The example in figure 4 shows that a small 4° bank angle away from the inoperative engine ensures a lower – safer – VMC(A) than when the wings are kept level (0° bank) and, in addition, results in a zero sideslip angle, hence the lowest drag and hence, maximum climb performance to be achieved when an engine fails or is inoperative. Jan Roskam: "The VMC(A) value ultimately used ties takeoff performance to engine-out controllability".
The VMC(A) for wings level (in this example 100 kt) is higher than the AFM-published VMC(A). Therefore, the pilot, after engine failure or during continuing a flight while an engine is inoperative, should maintain the exact bank angle that was used to design the vertical tail and at which the drag is minimal (in the example of figure 4: 4° away from the inoperative engine), as long as the asymmetrical thrust is maximum and the airspeed is as low as VMC(A). The published VMC(A) is valid for steady straight flight only, while maintaining a fixed small bank angle away from the inoperative engine. The required bank angle is published with VMCA and VMC in the AFM. When turns are unavoidable, the thrust can be reduced temporarily below maximum to reduce the thrust yawing moment which reduces the 'actual' VMC(A). 'Actual' VMC(A) is the VMC(A) that the pilot experiences in-flight, with the actual values of factors having influence on VMC(A), see the next paragraph.
Manufacturers may decide not to use a small bank angle for sizing the tail and determining VMC(A), and accept a higher than minimum drag, which is allowed unless the airplane violates the minimum climb requirements in the aviation regulations, because of the increased sideslip, hence drag. The effect of bank angle on VMC(A), as shown in figure 4, does not change.
Factors having influence on VMC(A)
Any factor that has influence on the balance of forces and on the yawing and rolling moments after engine failure might also affect VMCs. During designing the vertical tail and during flight-tests to measure VMC(A) and VMCL in-flight (explained below), the worst case of all factors that have influence on these VMCs were used to ensure that the VMCs that are published in the AFMs are highest - safest, whatever the actual configuration of the airplane is. The worst-cases of some of these factors are:
- low weight (smallest side force W•sin ø),
- center of gravity aft (shortest moment arm to the rudder) and laterally into the dead engine (larger thrust yawing moment),
- critical engine inoperative (largest thrust yawing moment),
- low altitude and low temperature (highest asymmetrical thrust yawing moment), and
- propeller wind-milling if not auto-feather equipped (propeller drag that increases the thrust yawing moment).
Three often used, though less influential, factors such as P-factor, critical engine and sudden failure, are briefly discussed first.
- P-factor refers to the lateral shift of the propulsion vector (P-vector) in a rotating propeller disc due to the increase of the angle of attack as the airspeed decreases, resulting in an increased yawing moment, that has to be counteracted by increasing the rudder input for maintaining steady straight flight.
- Critical engine. Due to the P-factor, there is a difference in the remaining yawing moments after failure of the left or the right (outboard) engine when both propellers rotate in the same direction. An engine can also be called critical when it is the only engine that drives a hydraulic pump for augmenting flight controls. Refer to the critical engine article. The published VMC(A) is measured while the critical engine fails or is inoperative. However, actual, yet a little lower VMCs also exist when anyone of the other engines fails or is inoperative. Hence, pilots do not have to know about the criticality of an engine.
- Sudden failure. The dynamic effects of a sudden failure do not necessarily have greater adverse effect on controllability than an inoperative engine during continuing the flight, including turning, while an engine is inoperative. During flight-testing both the dynamic and the static VMC(A)s are determined. Refer to the VMC(A) testing paragraph below. Hence, pilots do not have to use "sudden failure", the published VMC(A) includes the effect of a sudden failure.
As explained, pilots do not need to know about these factors, because their effects are included in the AFM-published VMC data. However, pilots do need to know about the effect of throttle, rudder and bank angle, because inappropriate use of these controls might increase the actual VMCs above the published and indicated VMCs and render the airplane uncontrollable:
- Throttles. VMC(A) and VMCL are determined with maximum thrust on the engine opposite of the inoperative engine. A lower (asymmetrical) thrust level reduces the actual VMC(A) to a safer level, actual VMC(A) being the VMC(A) that the pilot experiences in-flight, with the actual values of factors that have any influence.
- Rudder. A rudder input less than maximal (partial rudder), when the asymmetrical thrust is high, requires a higher airspeed for maintaining the balance of side forces for steady straight flight; actual VMC(A) is higher than the published VMC(A).
- Bank angle. A small bank angle away from the inoperative engine is required for smallest possible sideslip and lower actual VMC(A), as explained above. Keeping the wings level, or into the dead engine, increases VMC(A) to some 'actual' level and increases the sideslip. If the higher 'actual', though not-indicated, VMC(A) increases above the actual calibrated airspeed, control will be lost.
Not maintaining steady straight flight while banking a few degrees away from the inoperative engine, when the asymmetrical thrust is maximal, is also called an inappropriate crew response to propulsion system malfunction (ICR/PSM).
VMC(A) testing
The experimental test crew, usually existing of a test pilot and a flight test engineer will determine VMC(A) in-flight while all factors that have influence on VMC(A) are at their worst case values (i.e. aft center of gravity, low weight, critical engine inoperative, etc.), the result being a standardized worst-case VMC(A). The test technique is to shut down the critical engine and set the opposite engine at maximum thrust at an airspeed well above the pre-flighttest analyzed VMC(A). Then the airspeed is slowly reduced while maintaining straight flight (rudder) and wings level (ailerons). When the heading no longer can be maintained with maximum rudder (or banking can no longer be controlled using ailerons), the actual calibrated airspeed is VMC(A) with zero bank angle, i.e. wings level. Then, the bank angle is allowed to increase to the same bank angle that was used to design the vertical tail (usually 5° away from the inoperative engine) which allows the airspeed to be further reduced until again the heading or bank angle no longer can be maintained. The actual calibrated airspeed at that time is the static VMC(A) of the airplane (figure 4). The airspeed decrease from wings-level is approximately 8 kt for a small twin, up to 30 kt for a big four-engine jet. This in-fact means that when at VMC(A) the wings are rolled back to wings level, the heading cannot be maintained; control will be lost because the actual VMC(A) with wings level is (8 - 30 kt) higher.
In addition, the test crew determines the dynamic VMC(A): the critical engine is suddenly shut down to investigate the dynamic effects at several airspeeds down to the airspeed at which the heading change is maximum 20°, the bank angle does not exceed 45°, no dangerous attitudes occur and no exceptional piloting skill is required (i.a.w. FAR/ CS-23, 25 § 149).
The actual calibrated airspeed at that instant is the dynamic VMC(A) of the airplane. The highest of the static and dynamic VMC(A) will be published in the limitations section of the AFM as the VMC(A) of the airplane. Usually this will be the static VMC(A).
The flight-test techniques for measuring all VMCs of an airplane can be found in flight test guides for part 23 and part 25 airplanes, issued by the FAA and by EASA. The major test pilot schools in the world teach experimental flight-testing, including flight-tests to measure VMCs.
VMC(A) testing is always performed at a safe altitude of at least 5000 ft above ground level. The test data (acquired at several altitudes) is extrapolated and reduced to sea level or ISA before publishing in AFMs. Some manuals publish VMC(A) data in tables for several altitudes and temperatures to facilitate hot and high operations more accurately.
A manufacturer might decide to design the vertical tail and determine VMCs for level wings only. In any case, the bank angle for which the published VMCs are valid to be presented with the VMC data.
The published VMC(A) is a standardized VMC(A), refer to a few of the test conditions mentioned above and in applicable papers. Measuring all VMCs of an airplane for all configurations would leave pilots with huge data manuals. Publishing a worst case, standardized VMC(A) is always safe, provided the pilot is aware of the applicable limitations for the standardized VMC(A) to be valid.
Definition of VMC(A)
For airplane design engineers: | For pilots: |
---|---|
FAR and EASA/CS 23 and 25 § 149 and equivalent present the definition of VMC (is VMCA) for the design and certification of multi-engine airplanes:
Minimum control speed is the minimum flight speed at which the airplane is controllable with a bank angle of not more than 5° when the critical engine suddenly becomes inoperative and the remaining engine is operating at takeoff power. This definition is correct for tail design engineers and for certification but is often inappropriately copied into pilot manuals, because it contains elements that are neither appropriate, nor applicable to operational pilots. |
Once the airplane is designed and built, the selected tail size imposes control limitations on, i.e. conditions to, pilots. The VMC(A) definition for use by pilots is therefore different from the VMC(A) definition for tail design engineers and for certification, and could be:
VMC(A) is the minimum speed for maintaining control after a sudden engine failure and for maintaining steady straight flight while an engine is inoperative when the corresponding opposite engine is providing maximum thrust, provided a bank angle is being maintained of (3° – ) 5° away from the inoperative engine and the rudder is used up to maximum to maintain steady straight flight. The exact required bank angle (also used to size the vertical tail) is to be provided by the airplane manufacturer with the VMC data. In addition, the manufacturer should specify the configuration (flaps, etc.) for which this, and other published VMCs is valid. VMC(A) is defined for steady straight flight only, while banking a few degrees away from the inoperative engine and not for turns. |
Display of VMC(A)

On the airspeed indicator of part 23 twin-engine airplanes of less than 2722 kg or 6000 lb maximum weight, the standardized AFM-published VMC(A) is indicated by a red radial line, in the example of figure 5 at 80 kt, in accordance with § 23.1545 of aviation regulations.

In commuter part 23 airplanes of more than 2722 kg or 6000 lb maximum weight, the placard shown in figure 6 is to be installed in full view of pilots of to comply with § 23.1563 of aviation regulations.
Heavier part 25 airplanes now use VR and V2, instead of VMC(A). At low gross weight however, VMC(A) and the conditions for VMC(A) to be valid may still be of concern.
VMC(A) applies before and after failure of any of the engines, critical or non, and for any configuration or as specified by the manufacturer, during takeoff, but also during the remainder of the flight.
VMCA1 and VMCA2
Four- or more engine airplanes not only have a VMC(A), also called VMCA1 where the critical engine alone is inoperative (n-1), but also a VMCA2 that applies as minimum control speed when one engine is already inoperative. VMCA2 is determined while both the critical engine and the engine inboard of it on the same wing are inoperative (n-2), which is the worst case. Civil aviation regulations (FAR, CS and equivalent) no longer require a VMCA2 to be determined, although it is still required for military airplanes with four or more engines. On turbojet and turbofan airplanes, the outboard engines are usually equally critical. Three-engine airplanes such as the MD-11 and BN-2 Trislander do not have a VMCA2; a failed centerline engine has no effect on VMC.
When two opposing engines of airplanes with four or more engines are inoperative, there is no thrust asymmetry, hence there is no rudder requirement for maintaining steady straight flight; VMC(A)s play no role. There may be less power available to maintain flight overall, but the minimum safe control speeds remain the same as they would be for an aircraft being flown at 50% thrust on all four engines.
Failure of a single inboard engine, from a set of four, has a much smaller effect on controllability. So long as speed is maintained at or above published VMC(A), as determined for the critical engine, safe control can be maintained.
Limitations that apply to VMC(A)
The consequence of the tail design method is that the (small) bank angle requirement for maintaining control and performance while an engine is inoperative needs to be communicated to the pilots. They need to be made aware that the vertical tail is not large enough for maintaining control when the small bank angle is not being maintained (while the thrust is high and the rudder is maximum for maintaining the heading), not even at airspeeds above the published (and red-lined) VMC(A) (figure 5). Not maintaining the small bank angle increases the sideslip, hence drag, decreasing the performance, but also increases VMC(A); loss of control and/or loss of climb performance are very likely to occur. The vertical tail is not sized large enough to maintain control during turns at airspeeds as low as VMC(A) while the thrust of the opposite engine is maximum.
Safe turns at low speed while an engine is inoperative
For turning safely while the asymmetrical thrust is high, altitude should be gained first during steady straight flight to allow for some altitude loss during turns because of the increased sideslip (drag) during turns. It is safer to reduce the thrust a little during the turns to reduce the thrust yawing moment and required rudder and therewith reduce the 'actual' VMC(A). In addition, when the thrust is asymmetrical, a long straight-in approach should be considered, rather than a tight final turn during which the thrust might have to be increased to maximum and control will be lost because actual VMC(A) increases above the calibrated airspeed (figure 4).
On-line engine-out trainer
The University of North Dakota presents an on-line engine-out trainer to demonstrate the effects of several variables on VMC(A) and provides additional clarification in text blocks.
Minimum control speed on the ground – VMCG
When an engine fails during the takeoff run, the thrust yawing moment will force a displacement of the airplane on the runway. If the airspeed is not high enough and hence, the rudder generated side force is not powerful enough, the airplane deviates from the runway centerline and might even veer off the runway if the asymmetrical thrust setting is maintained. The airspeed at which the airplane, after engine failure, deviates 9.1 m (30 ft) (!) from the runway centerline, despite using maximum rudder but without the use of nose wheel steering, is called the minimum control speed on the ground (VMCG). The propeller, if applicable, is in the position it automatically achieves after engine failure. VMCG is used to calculate V1, the takeoff decision speed, i.a.w. § 23.107/ § 25.107 of aviation regulations part 23 and part 25.
Definition of VMCG
VMCG is the lowest speed at which the takeoff may be safely continued following an engine failure during the takeoff run.
Minimum control speed during approach and landing – VMCL
The minimum control speed during approach and Landing (VMCL) is similar to VMC(A), but the airplane configuration is the landing configuration. VMCL is defined for part 25 airplanes only in civil aviation regulations. However, when maximum thrust is selected for a go-around, the flaps will be selected up from the landing position, and VMCL no longer applies, but VMC(A) does. The bank angle limitation applies for VMCL as well as for VMC(A), although an additional requirement for roll control applies, see the next paragraph.
Definition of VMCL
VMCL is the airspeed at which it is possible to maintain control of the airplane in the landing configuration when an engine fails or is inoperative. In addition, lateral control at VMCL is adequate to roll the airplane from straight flight through an angle of 20° away from the inoperative engine in not more than five seconds.
VMCL1 and VMCL2
Four- or more engine airplanes not only have a VMCL, also called VMCL1, for the critical engine inoperative (n-1), but also a VMCL2 for the critical engine and the engine inboard of it inoperative on the same wing (n-2).
Testing VMCL
The flight-tests for determining VMCL are similar to the flight testing for VMC(A) explained above, with the exception of the (landing) configuration of the airplane and the roll rate requirement. This test is also performed at a safe altitude.
Safe single-engine speed – VSSE
Shutting down an engine suddenly in-flight at lower airspeeds might turn out to become very dangerous. Therefore, manufacturers, in accordance with aviation regulations § 23.149 define a VSSE, a speed at which the critical engine can be safely shut down for training and demonstration purposes of low speed engine-out flight and VMC(A), and publish this speed in the limitations section of the AFM. If VSSE is safe for rendering the critical engine inoperative, it is certainly also safe for rendering any other engine(s) inoperative (the actual VMC(A) is lower). Maintaining steady straight flight while banking a few degrees away from the inoperative engine might also be required to continue the flight safely.
Climb performance while an engine is inoperative – VYSE and VXSE
The presented performance data is valid only when the V speeds for achieving maximum performance while an engine is inoperative, i.e. while single-engine with a two-engine airplane: VYSE for maximum rate of climb and VXSE for maximum angle of climb. The airspeed for maximum single-engine rate of climb VYSE is indicated by a blue radial line on the airspeed indicator, in the example of figure 5 at 105 kt. At this airspeed, the sideslip, hence drag is minimal and the remaining climb performance is maximal, provided a small bank angle is being maintained away from the inoperative engine. This bank angle should be included in the legend of the climb performance chart - one engine operating, because not maintaining this bank angle renders the presented performance data invalid; the airplane might not even be able to maintain altitude if the bank angle is not maintained. The bank angle is smaller than 5°, because the presented performance data requires airspeed VYSE, the blue line speed, which is higher than VMC(A). The vertical tail is of course more effective at this higher speed; the required bank angle for zero sideslip can be smaller for equilibrium of forces and moments (side force W•sin ø, figure 3). Keeping the wings level or turning while an engine is inoperative at VXSE or VYSE means increased sideslip and loss of performance; the altitude cannot be maintained on most multi-engine airplanes.
References
- ^ Federal Aviation Administration, USA. "Federal Aviation Regulations (FAR)". Part 23 and Part 25, § 149. Retrieved May 15, 2016.
- ^ European Aviation Safety Agency. "Certification Specifications (CS)". CS-23 and CS-25, § 149. Retrieved Oct 28, 2013.
- ^ Roskam, Dr. Jan (1989). Airplane Design Part VII. Lateral-Directional Stability and Control Characteristics for Steady Straight Line Flight, Chapter 5. DARcorporation. p. 286.
- ^ Roskam, Dr. Jan (1997). Airplane Design Part II. Preliminary Configuration Design and Integration of the Propulsion System, Chapter 11. DARcorporation. p. 267.
- ^ USAF Test Pilot School, Edwards Air Force Base, CA, USA (1992). Engine-Out Theory, Chapter 11 (PDF). Retrieved May 15, 2016.
{{cite book}}
: CS1 maint: multiple names: authors list (link) - ^ Empire Test Pilots' School, Boscombe Down, UK. Flight on Asymmetric Power.
{{cite book}}
: CS1 maint: multiple names: authors list (link) - ^ USNaval Test Pilot School. Flight Test Manual USNTPS-FTM-No. 103, Fixed Wing Stability And Control, Theory and Flight Test Techniques, Chapter 6 – Asymmetric Power Flying Qualities (PDF). Retrieved May 15, 2016.
- ^ Military Specification MIL-F-8785C, superseded by MIL-STD-1797. Flying Qualities of Piloted Airplanes.
{{cite book}}
: CS1 maint: numeric names: authors list (link) - Horlings, Harry. "The Effect of Bank Angle and Weight on the Minimum Control speed VMCA of an Engine-out Airplane" (PDF). AvioConsult. Retrieved Oct 28, 2013.
- Roskam, Dr. Jan (1989). Airplane Design Part VII. Lateral-Directional Stability and Control Characteristics for Steady Straight Line Flight, Chapter 2. DARcorporation. p. 28.
- Federal Aviation Administration, USA. "Advisory Circular 23-8C, Flight Test Guide" (PDF). Retrieved Oct 28, 2013.
- Federal Aviation Administration, USA. "Advisory Circular 25-7B. Flight Test Guide" (PDF). Retrieved Oct 28, 2013.
- European Aviation Safety Agency. "Certification Specification 23 Flight Test Guide" (PDF). Retrieved Oct 28, 2013.
- Horlings, Harry. "Control and Performance during Asymmetrical Powered Flight" (PDF). AvioConsult. Retrieved Oct 28, 2013.
- On-line Engine-out Trainer, University of North Dakota, USA, Retrieved Oct. 28, 2013.